NanoAndMore USA does not ship to your country
Ultra High Frequency AFM Probes
High speed scanning AFM (HSS-AFM, HS-AFM) is mode of AFM operation in which scanning is performed at much higher speeds than those achieved in conventional AFM. This enables shortening image acquisition times dramatically - from tens of minutes down to seconds. More importantly, HS-AFM opens the possibility for investigation of dynamic phenomena at the nanoscale. For example, HS-AFM is commonly used in liquid environments to study biological molecules such as DNA and proteins during their functional activities, structural changes occurring on the surfaces of live cells, dynamic processes in synthetic polymers, electrochemical reactions and crystallization of organic and inorganic materials. The visualization of dynamic processes makes it easier to analyze and understand them.
The general principle of HS-AFM operation is not very different from the one of tapping mode AFM. While the AFM tip scans the sample surface the Z-feedback system works to maintain the tip-sample interaction force constant. In amplitude modulation mode, the AFM cantilever is excited at its primary resonance frequency. As the sample height under the AFM tip changes, the AFM cantilever oscillation amplitude changes and the feedback loop controls the Z-piezo, moving the AFM probe up and down in order to maintain constant oscillation amplitude.
Due to the finite reaction time of all involved elements, it takes time for the feedback loop to react to a height change and return the AFM cantilever to the setpoint. Since the AFM tip velocity in HS-AFM is much higher than that in standard tapping mode, the delay in the response of each element is much more critical. In HS-AFM, all elements are optimized for fast response. In addition, various methods are implemented to suppress the vibrations which are often generated during high speed scanning.
The Z-scanner and the AFM cantilever are mechanical devices and their response is slow compared to that of the electronics devices. The AFM cantilever response time is proportional to its quality factor and inversely proportional to its resonance frequency. Considering the fact that the quality factor is low in fluids, the resonance frequency must be high to reduce response time. This requirement is fulfilled by advances in the manufacturing process leading to miniaturization of the AFM cantilevers. Small AFM cantilevers with length in the range of 10 micrometers, width of 2 micrometers and thickness of approx. 100 nanometers are implemented. They have resonance frequencies in the range of 400 to 800 kHz, low force constants in the range of 0.1 to 0.2 N/m and quality factors of 1 to 2 in water.
The response time of the Z-scanner is approximately determined by the Z-piezoactuator. It is proportional to the phase delay at the driving frequency and inversely proportional to this driving frequency. For a Z-range of 0.5 to 1 micrometer frequencies in the range of 200 kHz can be achieved. Finally, techniques for fast AFM cantilever deflection-amplitude conversion are developed. The amplitude at multiples times during a single AFM cantilever oscillation cycle.
When the feedback loop response time is not sufficient for a given tip velocity, the interaction force between the AFM tip and the sample is increased relative to the setpoint when the AFM tip climbs the slope of a high feature and the interaction force is decreased when the AFM tip climbs down. As the tip velocity is increased further, the AFM tip taps harder and harder on up-hills, resulting in tip and/or sample damage, and completely loses contact with the surface on down-hills, resulting in image artefacts. The latter effect has been remedied by the development and implementation of feedback controllers with automatic gain adjustment.
A phase delay of 45⁰ is generally accepted as the threshold value beyond which the feedback error becomes unacceptable. The frequency at which this delay occurs is called the feedback bandwidth and this frequency is the most indicative parameter for the speed performance of the combination of the HS-AFM system plus the AFM cantilever. Feedback bandwidth values of up to 100 kHz can be achieved currently for AFM cantilevers with 1.2 MHz resonance frequencies.
HS-AFM has been gaining popularity since its inception. The number of HS-AFM related publications has been growing steadily since 1996. Most of these studies have been performed mainly to test the performance and analyze the potential of the developed HS-AFM systems. They can be categorized as conformational changes of proteins in their functional activities; processes of aggregation, self-assembly and disassembly; structural transitions and fluctuations; associative and dissociative dynamic interactions; enzyme reactions; diffusion; cellular morphological changes and dynamics of proteins on cell surfaces and dynamic processes in artificial systems made of biological materials. In addition, HS-AFM has been implemented in force spectroscopy measurements with high loading rates.
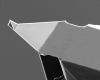
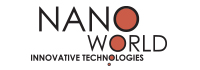
Ultra High Frequency AFM Probe with Tip at the Very End of the Cantilever
Tip Shape: Arrow
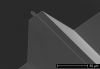
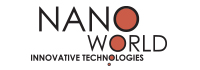
Tip Shape: Cone Shaped,EBD
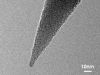
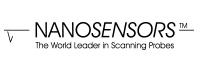
Tip Shape: Circular symmetric
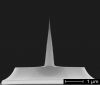
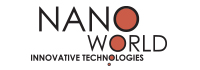
Tip Shape: Cone Shaped,EBD
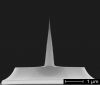
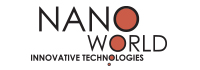
Tip Shape: Cone Shaped,EBD
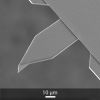
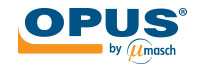
Gold Coated Ultra High Frequency AFM Probe with AFM Tip at the Very End of the AFM Cantilever
Tip Shape: Optimized Positioning
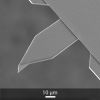
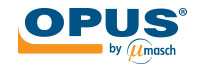
Ultra High Frequency AFM Probe with AFM Tip at the Very End of the AFM Cantilever
Tip Shape: Optimized Positioning
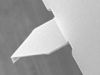
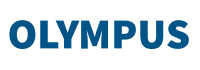
Tip Shape: Optimized Positioning
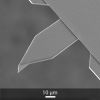
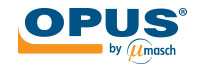
Ultra High Frequency AFM Probe with AFM Tip at the Very End of the AFM Cantilever
Tip Shape: Optimized Positioning
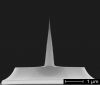
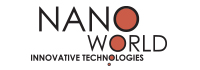
Tip Shape: Cone Shaped,EBD
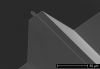
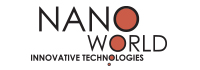
Tip Shape: Cone Shaped,EBD
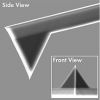
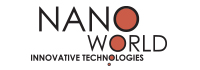
Tip Shape: Arrow
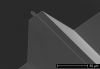
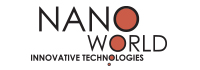
Tip Shape: Cone Shaped,EBD
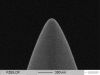
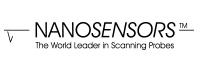
Tip Shape: various